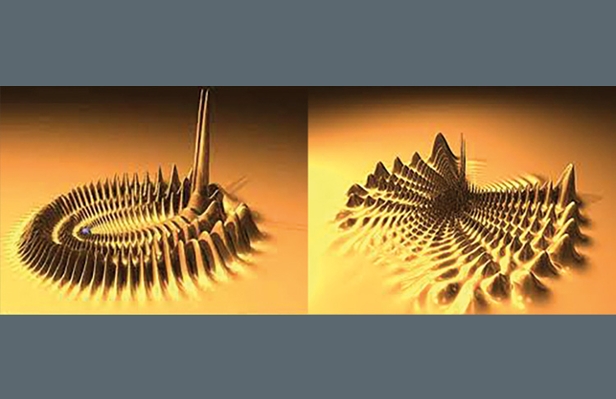
Professor Chris Greene Elected to the National Academy of Sciences
By Elizabeth Gardner
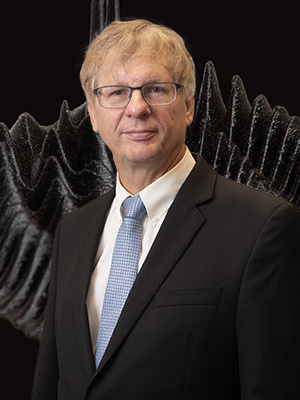
A picture of the trilobite molecule graced the textbook cover of "Physical Chemistry" even before the molecule had been proven to exist.
"I guess they had confidence in me and my co-authors," Chris Greene says with a modest chuckle. The confidence was warranted. In 2019 Greene was elected as a member of the National Academy of Sciences — one of the highest honors given to a scientist in the United States.
Greene, Purdue's Albert Overhauser Distinguished Professor of Physics, is a leader in the rapidly growing field of atomic, molecular and optical physics, or AMO physics. The trilobite Rydberg molecule he predicted was experimentally proven in 2008 and is one example of his many scientific achievements.
"Chris exemplifies the powerful, world-changing research done at Purdue University," Purdue President Mitch Daniels said when the academy announced the news. "Membership in the National Academy of Sciences is one of the pinnacles of distinction for a scientist. He has long established himself as a leader in his field, and we couldn't be more proud to have him as part of our faculty."
Greene is perhaps best known for a theory of an unusual binding mechanism in ultracold quantum gases and the existence of huge Rydberg molecules, electronically excited molecules that behave in unique ways and can exhibit exaggerated or relatively "giant" sizes. The two-atom rubidium Rydberg molecules that Greene predicted with Alan Dickinson and Hossein Sadeghpour are known as the trilobite molecule and butterfly molecule — named for their electron probability models’ resemblances to these creatures.
Greene's research has largely focused on few-particle quantum systems, involving only one or two atoms with few electrons, and the dynamics of atomic bonds. Such systems are far more complicated than those involving many particles because averages and approximations of gross behavior no longer work. With only a few particles involved, the degrees of freedom impacting each must be thoroughly understood. Greene has been building a toolkit of methods to determine what is happening in these systems that have been considered too complicated to solve in many cases.
"We want to pull out the key insights and to identify, out of all the zillions of ways the system could move, the crucial motions and crucial energies and positions where things change the seat of reactivity," he says. "There's so much satisfaction in seeing this toolkit that I've been working on since I was a graduate student growing and being able to tackle harder and harder problems that looked unsolvable just a decade ago."
Understanding such fundamental physics is essential to developing methods for the control and manipulation of these systems that could lead to a wide variety of applications. Greene and his teams have solved problems with applications in astrophysics, ultracold quantum gases, chemical physics, nuclear physics, and atomic physics with potential relevance to quantum information and computing.
I think AMO physics was a bit player in the field of physics for years, and physicists in other areas thought ‘That’s all old stuff and has been understood since the 1940s,’” Greene says. “But now, it is one of the fastest-growing areas of the American Physical Society. Now they understand that through AMO we are developing ways to have exquisite control of atoms and molecules — experimentalists have been able to make an atomic clock with a 10-18 level of accuracy. There have been astonishing achievements. It’s just unbelievable how far the AMO toolkit has taken both technological and fundamental science capabilities.
John Finley, head of Purdue's Department of Physics and Astronomy, says Greene has made significant contributions to both the field and Purdue's department.
"Professor Greene has made important contributions to theoretical AMO physics in the areas of dissociative recombination, ultracold matter and high-harmonic generation," Finley says. "He also predicted the existence of 'trilobite' long-range molecules, so called because of the unique pattern formed by their electron clouds. Professor Greene is the foundation of our AMO group, and this area is a fertile ground of alternate ways to explore unusual quantum states in nature. These unusual states are at the heart of many of the ideas behind quantum computation."
Greene has previously been recognized for his contributions to research with the I.I. Rabi Prize in Atomic, Molecular and Optical Physics in 1991; the Davisson-Germer Prize in 2010; and the Hamburg Prize for Theoretical Physics in 2013.
He is the first full-time faculty member from Purdue's Department of Physics and Astronomy to be elected to the academy since the late Albert Overhauser was elected in 1976, and he joins four Purdue colleagues as current members or foreign associates of the academy: R. Graham Cooks, the Henry Bohn Hass Distinguished Professor of Chemistry; H. Jay Melosh, Distinguished Professor of Earth and Atmospheric Sciences and Physics; Ei-ichi Negishi, Nobel laureate and the Herbert C. Brown Distinguished Professor of Chemistry; and Jian-Kang Zhu, Distinguished Professor of Plant Biology.