Home
Research Interests
Graduate Research
Undergraduate Research
Publications
Quantum Movies Class Info
|
Quantum Simulator and Computer Projects
For the most part, we do not directly perform calculations of
quantum simulators or computers in the sense of creating or
investigating algorithms, testing various protocols, etc. Our main
interest is in studying the physics of the different parts of quantum
simulator or computer platforms with a concentration on effects that
are typically left out of the idealized studies. We also develop the physics of the
components of different platforms so that quantitatively accurate
interactions within a quantum simulator or computer can be predicted.
Below is a brief
description of results in two recent publications. These investigations
are good examples of our interests. The first highlights the role that
recoil of atoms when a single photon interacts with an array can be
substantially more problematic than expected. The second investigates
the infidelity on a particular kind of gate due to many different
experimental parameters.
Deepak A. Suresh and F.
Robicheaux, “Photon-induced atom recoil in collectively interacting
planar arrays,” Phys. Rev. A 103,
043722 (2021). PDF
(655 kB) (data for figures at https://doi.org/10.4231/HHNZ-SP39)
In this paper, we studied how atoms in an array recoil due to the
emission or absorption of a photon. This is an important question
because an atom that recoils can have its center-of-mass motion
entangle with internal degrees of freedom which leads to unwanted
decoherence in the operation of quantum simulators or computers. This
is not a simple question because a photon can distribute the recoil
over many atoms. We also found cases where a single photon could lead
to 1000X the expected recoil energy deposited into a single atom. Also,
the interference between the incident and scattered photon field can
lead to nontrivial patterns of energy deposition in the array.
The left image shows the electric field for a proposed cavity composed
of atoms on a grid; the bright spots are the positions of the atoms
which enhance the intensity in their neighborhood. The proposal did not
account for the possible recoil of the atoms in the array. The right
image shows the recoil energy for an example cavity. Note that
the center atom gains approximately 1000 recoil energies before
the photon is lost from the cavity. This high recoil results from the
photon bouncing many times between the mirrors constructed from the
atom arrays.
These images are for light perpendicularly incident on an 11X11 array
of atoms. The left plot shows the probability for each atom in the
array to be excited by the photon. The right plot shows the amount of
recoil energy deposited into each atom per photon incident on that
atom. Although each photon can only interact once with the array, many
of the atoms gain more than 2 recoil energies. Also, there is a highly
nontrivial pattern of energy deposition which roughly matches the
pattern of excitation of the array.
F.
Robicheaux, T.M. Graham, and M. Saffman, “Photon-recoil and
laser-focusing limits to Rydberg gate fidelity,” Phys. Rev. A 103,
022424 (2021). PDF
(642 kB) (data for figures at https://doi.org/10.4231/B7CM-VP26)
In this paper, we study several mechanisms that can decrease the
fidelity of a two atom gate that uses the Rydberg-Rydberg interaction
to entangle hyperfine states of a pair of atoms (see below). In
particular, we quantitatively study effects that lead to infidelities
from less than 0.0001 to 0.01. Examples of the processes we study
include: photon kick from a pair of counter-propagating laser
pulses, photon kick due to focussing of the light beam, residual kick
from the Rydberg-Rydberg interaction, etc.
This image is a cartoon of the type of gate investigated in this paper.
This is a gate that uses the Rydberg-Rydberg interaction between highly
excited atoms to entangle the hyperfine states being used as qubits. By
having many atoms in an array of optical traps, a quantum simulator or
computer can be constructed with this being the main 2-qubit entangling
gate.
Five Recent
Publications
A.
Trautmann, M. J. Mark, P.
Ilzhöfer, H. Edri, A. El Arrach, J. G. Maloberti, C. H. Greene, F.
Robicheaux, and F. Ferlaino, “Spectroscopy of Rydberg states in erbium
using electromagnetically induced transparency,” Phys. Rev. Res. 3, 033165 (2021). PDF
(1750 kB) (MQDT data at https://doi.org/10.4231/HK40-XM43)
F. Robicheaux,
“Calculations of long range interactions for 87Sr
Rydberg states,” J.
Phys. B 52,
244001 (2019). PDF
(489 kB)
F.
Robicheaux and Shihua Huang, "Atom recoil during coherent light
scattering from many atoms," Phys. Rev. A 99, 013410 (2019). PDF
(603 kB)
F.
Robicheaux, D.W. Booth, and M. Saffman, "Theory of long-range
interactions for Rydberg states attached to hyperfine-split cores,"
Phys. Rev. A 97,
022508 (2018). PDF
(678 kB)
M.T. Eiles, J.
Perez-Rios, F.
Robicheaux and C.H. Greene, "Ultracold molecular Rydberg physics in a
high density environment," J. Phys. B 49,
114005 (2016). PDF
(2010 kB)
|
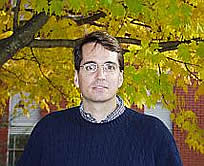
robichf[at]purdue.edu
284 Physics Building (Office)
+1 765 494 3029 (Office)
+1 765 494 0706 (FAX)
Mail:
Department of Physics
525 Northwestern Avenue
West Lafayette, IN 47907
Links:
Phyics
Department
Purdue University
ALPHA Project
|